Science is driving the demand for nanoscale resolution. Cryo-scanning electron microscopy (cryo-SEM), which combines the high-performance imaging of scanning electron microscopy (SEM) with cryogenic sample preparation techniques, allows scientists to investigate structures and materials in their native, hydrated state with image resolution now approaching 1 nm. Recent improvements in speed, ease of use, and imaging performance have transformed cryo-SEM from a highly specialized discipline to an accessible, routine laboratory technique with broad applications in the life and materials sciences.
Electron microscopy offers much higher resolution than optical microscopy, but is more complex to operate and imposes a variety of requirements for sample type and preparation. Optical microscopy can resolve structures down to the micrometer scale or slightly less and, in many cases, requires little or no sample preparation. Scanning electron microscopes can resolve features down to 1 nm, and transmission electron microscopes (TEMs) can see individual atoms with resolution as low as half an Angstrom (50 pm) in certain applications. There are a number of different types of electron microscopes, and they vary widely in their operational complexity and sample preparation requirements.
SEM constructs a virtual image by scanning a finely focused beam of electrons over the sample surface, measuring the intensity of the signals generated by interactions between the beam electrons and the sample atoms at each point in the scanning pattern, and mapping that intensity to a corresponding point in a gray-scale image. SEM may be regarded primarily as a technique for looking at surfaces, since the imaged signals originate at or relatively close to the sample surface. SEM resolution is determined primarily by the size of the spot formed by the beam on the sample surface and the size of the volume below the spot within which detectable signals are generated.
TEM, and closely related scanning transmission electron microscopy (STEM), create images from information carried by high-energy beam electrons that have passed through the sample. Although these techniques are capable of higher resolution, their need for samples thin enough to transmit electrons (generally less than 100 nm thick) significantly increases their operational complexity and sample preparation requirements.
Of the various types of electron microscopes, the SEM is arguably the easiest to use and has the least extensive requirements for sample preparation. Subnanometer resolution, large depth of field, and high sensitivity to surface topography make SEM an ideal technique for investigating complex surfaces. Although SEM cannot offer the sub-Angstrom resolution of transmission and scanning transmission electron microscopy, neither does it need the difficult-to-prepare, ultrathin samples required by those techniques.
Cryo sample preparation
All electron microscopes must operate with some level of vacuum in order to minimize the scattering of electrons by gas molecules. This imposes a number of constraints on the sample and usually requires certain sample preparation procedures. To be compatible with the vacuum, the sample must not outgas or otherwise degrade the vacuum environment, and the structures of interest in the sample must not be modified by the presence of the vacuum. Furthermore, in nonconductive samples, some provision must be made to dissipate charge deposited at the sample surface by the electron beam—generally by creating a path to ground through a conductive coating. Therefore, sample preparation for SEM typically consists of some combination of chemical or physical drying, fixing, and coating procedures. Unfortunately, all of these have the potential to introduce artifacts and interference into SEM imaging and analysis.
Cryo-SEM uses low temperatures to prevent the evaporation of volatile components such as water. In so doing, it provides compatibility with the vacuum environment and preserves the natural structures of the sample, which are often supported by the presence of these volatile components. This is particularly true for samples in the life sciences, where structures will retain their original conformation only in their fully hydrated state.
Coatings remain important in cryo-SEM. In addition to providing a conductive pathway for the dissipation of charge, they can actually enhance the surface detail observable in the SEM image. Contrast in an SEM image (using the secondary electron signal) results primarily from variations in local topography. In fact, this sensitivity to topography is one of the primary advantages of SEM. Image interpretation is quite intuitive. Protruding edges appear bright, and depressed features are dark, so that the image looks much like an aerial photograph.
Biological samples are usually composed primarily of relatively light elements—carbon, hydrogen, and oxygen. The depth to which the beam electrons penetrate the sample, and therefore the size of the interaction volume and the resolution of the image, is a function of beam energy and sample composition.
Higher energy and lighter element composition allow deeper penetration and degrade image resolution. The presence of a thin, heavy element coating, such as a metal, can reduce beam penetration and enhance surface detail in images of light element samples.
It follows from this discussion that beam energy also plays a significant role in image quality. Generally, lower beam energy results in less penetration and better surface detail. However, this assumption must be moderated by the effect of beam energy on the electron optical system’s ability to focus the beam into a small spot. Chromatic aberration in the magnetic lenses used in all electron microscopes limits the minimum size of the spot when operating at low accelerating voltages, and performance worsens as the accelerating voltage decreases. Thus, there is some ideal combination of coating thickness and composition and accelerating voltage that will provide optimal image quality. In this context, new technologies that reduce the effects of chromatic aberration, such as monochromators that limit the energy differences among beam electrons, can be expected to provide significant benefits in cryo-SEM applications.
The primary source of interference and artifacts in cryo-SEM images is contamination. Because the sample is very cold, contaminants from the environment collect readily on the sample surface. Contaminants may originate in the microscope, such as hydrocarbons from oils used in the vacuum system, or from the sample itself, such as water molecules that sublimate from the sample surface. Contamination can be reduced by the use of anticontamination devices, typically a surface or enclosure positioned near the sample and held at the same or lower temperature to capture contaminants away from the sample surface. The regular use of a plasma cleaning system in the sample chamber can also help to reduce contamination.
Cryo-SEM is a dynamic process that involves constant balancing by the operator of competing processes occurring at the sample surface. Operator skill remains a primary factor in determining the quality of the results, particularly the operator’s ability to quickly and efficiently scan the surface, manipulate conditions, and acquire images of the rapidly changing sample. In this context, any simplification of operational control may contribute directly to the quality of the final result. For instance, a constant current capability that permits changes in accelerating voltage without changing the beam current gives the operator one less variable to manipulate.
All modern high-resolution SEMs use field emission guns (FEGs) to achieve the smallest possible beam diameter at usable beam current levels. An FEG SEM with a Schottky emitter (to provide beam stability) and a vacuum buffered column can be run at very low beam currents to minimize local heating and consequent damage to the specimen. This, along with fine continuous control of the accelerating voltage and beam current, allows precise setting of the instrument to suit the material being observed with minimal electron dose. It is thus possible to achieve high-resolution imaging on cryo samples at accelerating voltages of less than 6 kV. Such low voltages enable excellent topographical imaging at magnifications on the order of 100,000× or higher. Independent control of sample and cold trap temperatures prevents etching and condensation and permits long observation times without deterioration of the sample or constant correction of the cryo conditions. If condensation does occur, the sample can be sublimed within the microscope in situ, eliminating the need to return it to the cryo system.
Freezing is typically accomplished by mounting the sample on the transfer device and plunge freezing in liquid ethane or high-pressure freezing. The sample is then transferred under vacuum in a low-temperature state to the preparation chamber of the cryo system. Here, it can be sublimed or fractured and sputter-coated. Finally, it is transferred under vacuum and at a controlled temperature to the specimen chamber of the SEM for observation.
Natural surfaces
Samples that show extensive water-containing structures on the surface, such as plant leaves and petals, can be cryogenically frozen to retain the structures intact. Plunge-freezing with liquid nitrogen is sufficient for this type of sample. This type of sample is generally sublimed for 2 min in the preparation chamber at low temperature to remove natural surface ice, then sputter-coated for conductivity with a fine metal before transfer to the specimen chamber of the SEM.
Internal surfaces
In the cryo fracture technique, the sample is first cooled to a specified temperature, and is then fractured within the cryo chamber. Precise control of sample temperature is crucial since it controls the nature of the fracture. Cryo-fracturing a sample exposes internal structures for high-resolution observations. The exposed surface is typically sputter-coated in the cryo system before transfer to the specimen chamber.
Cryo sublimation
Even greater detail can often be revealed by carefully raising the temperature of a hydrated freeze-fractured specimen to a point at which water begins to sublime at a controlled rate (–90 °C to –100 °C). This etching process can be stopped at any time by again lowering the temperature. The technique can either be controlled in the specimen chamber or in the cryo chamber. The advantage of using the specimen chamber is that direct observations permit more precise control of the process.
Dehydration
Just as sublimation can be used to remove water from the sample surface, internal dehydration can occur if the sample is left long enough at a slightly higher temperature relative to the anticontaminator. The result is a completely dried sample, perhaps with some contraction of the outer surface but still showing important structure. In a related technique, the dried sample may be removed from the SEM and the sputtered coating floated off in a water bath. The result is a metal-sputtered replica of the original surface. This can then be collected by a support grid and examined with S/TEM for higher-resolution investigation.
Stabilization of low-melting-point solids
Suspensions, oils, fats, waxes, plastic polymers, and emulsified products are often either damaged by the electron beam or are not stable in the vacuum environment of an SEM. Such samples can be cryo-stabilized by freezing and transferred to a cryo preparation chamber under vacuum. There they can be fractured, sputter-coated with a conductive layer, and examined in the SEM. Fracturing such samples gives information on the distribution and size of the various phases and components within an individual formulation or product.
Application examples
Figures 1–6 demonstrate the ability of cryo-SEM to image surfaces in their natural states over a range of magnifications from 100× to 150,000×, with resolution in the high-magnification examples clearly down to the nanoscale level.
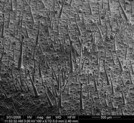
Figure 1 - Tobacco leaf surface (imaged at 100× magnification).
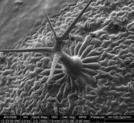
Figure 2 - Trichome on Arabidopsis leaf (imaged at 500× magnification).
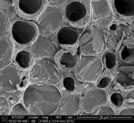
Figure 3 - Spiral core of dandelion stem (imaged at 3000× magnification).
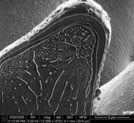
Figure 4 - Tobacco petal cross-sectioned, sublimed, and coated with PtPd (imaged at 12,000× magnification).
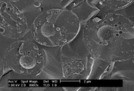
Figure 5 - Cryo-fractured yeast cell showing nuclei with pore caps (imaged at 8997× magnification).
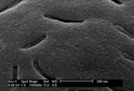
Figure 6 - Cryo-fractured yeast cell showing arrays of 10-nm transmembrane particles on the outer surface of the inner membrane (imaged at 150,000× magnification).
Conclusion
Cryo-SEM combines the high-resolution imaging of scanning SEM with cryogenic sample preparation techniques to allow researchers to observe nanoscale structures in their natural state with minimal artifacts or interference from sample preparation procedures. The improved speed, ease of use, and imaging performance of the current generation of SEMs and cryo preparation systems have made cryo-SEM accessible to almost any laboratory, supporting a wide range of applications in the life sciences and materials science. In response to the demands of modern science, cryo-SEMs are pushing the resolution capabilities of the technique down to the nanometer level and beyond.
Dr. Greiser is Product Marketing Manager, Life Sciences Division, FEI Co., 5350 N.E. Dawson Creek Dr., Hillsboro, OR 97124, U.S.A.; tel.: 503-726-7500; fax: 503-726-2570; e-mail: [email protected].