Common alkali and alkaline earth cations are not considered primary drinking water contaminants, but alkaline water can potentially interfere with water clarification and disinfection procedures and negatively impact water quality. High pH values can also cause corrosion of water mains and pipes, which in turn can result in water contamination.1 Calcium and magnesium, on the other hand, are essential minerals that have beneficial human health effects. Deficiency of any of these nutrients can cause adverse health consequences, which is why national and international bodies have introduced recommended daily intake levels. Whereas food is the primary source of calcium and magnesium, drinking water rich in these minerals also contributes to total intake. Nevertheless, high concentrations of dissolved calcium and magnesium in water results in increased water hardness and subsequent corrosion and scaling of delivery and drainage systems.2
Ammonia is a colorless, pungent gas that is highly soluble in water, where it exists in equilibrium between a molecular form associated with water and the ionized form (the ammonium cation, NH4+). Ammonia can enter environmental waters as a product of anaerobic decomposition of nitrogen-containing compounds or from waste streams containing ammonia. When dissolved in water, ammonia reacts to form ammonium, which at certain concentrations is toxic to aquatic and human life, with extreme ammonium levels causing coma and even death. Ammonium toxicity is pH and temperature dependent, increasing as pH and temperature decrease.3
In 2013, the U.S. EPA published national recommended water-quality criteria for the protection of aquatic life from the toxic effects of ammonia in freshwater, which emphasized the importance of ammonium monitoring.4 In Europe, Council Directive 98/83/EC specifies maximum acceptable values for ammonium in water intended for human consumption.5 National effluent standards are also uniformly applied in Japan to protect human health and the environment from the negative consequences of ammonium.6
Overcoming analytical challenges
Inorganic cations and ammonium have been analyzed by atomic absorption spectroscopy (AAS), inductively coupled plasma-mass spectroscopy (ICP-MS) and inductively coupled plasma-atomic emission spectroscopy (ICP-AES), as well as by electrochemical techniques such as polarography and anodic stripping voltammetry. Some methods are labor-intensive and difficult to automate, while suffering from spectral and chemical interferences, while other techniques are inappropriate for direct trace analyses in complex matrices or for studies on metal speciation.7
Some of these methods also lack multianalyte determination capability, meaning they are not suitable for the simultaneous measurement of inorganic cations and ammonium in the same sample. Instead, ammonium must be measured separately by a wet chemical technique, which may require a separate distillation step.8
In comparison to the analytical options listed above, ion chromatography (IC) has a number of advantages. Being a separation-based technique, it is capable of distinguishing between different metal-oxidation states. In addition, it can be used to determine stable metal complexes,9 and perform multianalyte analysis, enabling scientists to determine ammonium and inorganic cations in a single run.
The unique advantages of IC have been recognized by regulatory bodies. The U.S. EPA has approved a method for the analysis of ammonium, sodium, potassium, calcium and magnesium in rain, snow, dew, sleet and hail.10 Additionally, the International Organization for Standardization (ISO) has published a method for the simultaneous determination of dissolved alkali and alkaline earth cations, ammonium and manganese in water and wastewater using IC.11 The American Society for Testing and Materials (ASTM) also recommends an IC-based method for the simultaneous analysis of cations, which includes ammonium, sodium, potassium, calcium, magnesium and lithium in reagent water, drinking water and wastewaters.12
Rapid and reliable monitoring
Recent technological advancements have resulted in the introduction of compact, integrated high-pressure IC (HPIC) systems, which feature simplified, intuitive flow-path-based plumbing; thermally isolated temperature-controlled module compartments; and automated eluent generation. As a result, these instruments enable laboratories to address their analytical challenges, reducing time to equilibration; boosting sample throughput; and generating accurate, consistent and reproducible results.
IC systems can be equipped with high-capacity, cation-exchange columns that improve performance for trace-level determinations of cations in high-ionic-strength matrices by extending the linear range and resolving higher concentration ratios of sodium and ammonium. The use of 4-μm particle size columns produces more efficient separations, facilitating peak integration and permitting faster flow rates without compromising resolution. In combination, these features result in shorter run times and higher sample throughput.
While faster flow rates increase backpressure, the high pressure capability (up to 5000 psi with eluent generation) of current HPIC instruments extends the range of eluent flow rates that can be used, allowing the systems to take maximum advantage of smaller particle columns.
Laboratory testing
A Thermo Scientific Dionex Integrion HPIC system, equipped with a Thermo Scientific Dionex IonPac CS16-4μm column (Thermo Fisher Scientific, Sunnyvale, Calif.), was used to determine the concentrations of lithium, potassium, magnesium, calcium, sodium and ammonium in environmental water samples. Cation retention times varied with temperature, with potassium proving the most sensitive. This retention time variability can be further exploited to optimize selectivity among analytes, but a constant temperature should be maintained to ensure the best possible retention time reproducibility.
Cation standard solutions were initially run at the Quality Assurance Report (QAR) condition of 0.64 mL/ min, but to reduce the run time, flow was increased up to 1 mL/min (Figure 1). Even at this maximum recommended flow rate, the cation standards were baseline resolved. Good peak resolution (>1.5) at all flow rates was achieved due to the 4-μm particle column, which has greater peak efficiencies than standard, larger-particle-size columns. A flow rate of 0.9 mL/min was used for the remainder of the data presented here because it produced a relatively short run time while yielding sufficient resolution to quantify ammonium in the presence of a 10,000-fold excess of sodium. Using this flow rate and a 40 °C column temperature, calcium—the longest-retained cation—eluted within only 18 minutes, compared to 25 minutes at the QAR flow rate.
Figure 1 – Comparison of cation retention times at different flow rates.To establish the linear calibration range, the peak responses to concentration were determined using injections of calibration standards over a 2000-fold or greater concentration range. The high capacity of the 4-μm particle column resulted in a calibration curve that is linear over three orders of magnitude for most of the cations, except ammonium. A quadratic curve-fitting function extended the calibration curve for ammonium to a concentration of 40 mg/L. Coefficients of determination (r2) ranged from 0.9996 to 1.000. Suppressed conductivity allowed detection down to the low μg/L level with a 10-μL sample injection (Table 1). Depending on the ionic strength and nature of the sample matrix, lower detection limits may be achieved by injecting more sample.
Table 1 – Linear range and method detection limits (MDLs) for cations and ammonium
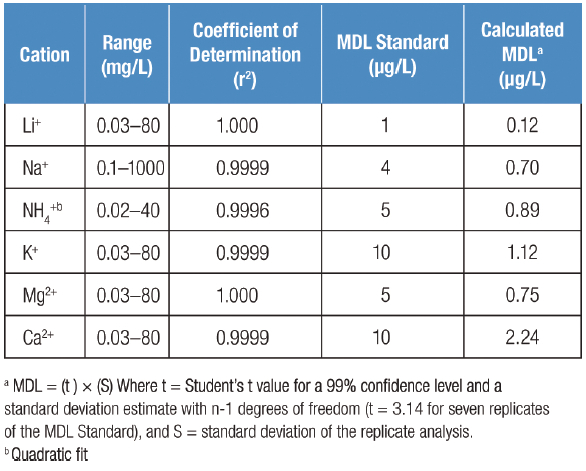
The HPIC system conveniently generated a high-purity methanesulfonic acid (MSA) eluent with very stable composition, leading to highly reproducible results. To validate the performance of the method, the levels of inorganic cations and ammonium in various environmental water samples were first determined. Samples were then spiked with the analytes at a level that was 50–100% of this amount. Spike recoveries of inorganic cations were ~100% in all matrices, ranging from 89 to 117%, which is within an acceptable range of 80–120% (Table 2).
Table 2 – Recovery of inorganic cations in water samples
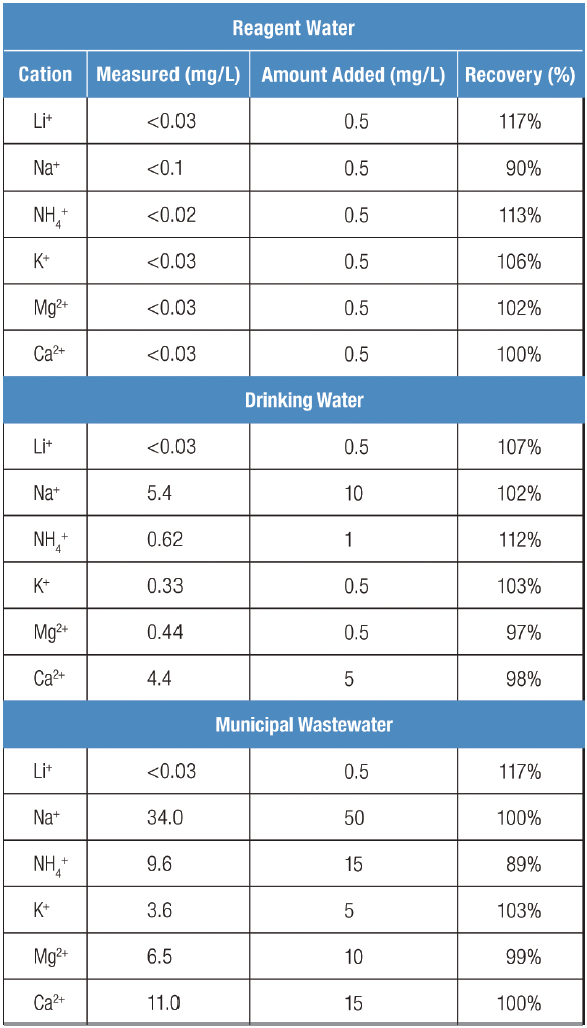
The high-capacity 4-μm column allowed for the analysis of environmental samples with a wide range of ionic strengths, without interference from the matrix (Figure 2). This column was effective for the determination of low concentrations of ammonium in the presence of high sodium (Figure 3). Alkanolamines can also be resolved under similar conditions.
Figure 2 – Determination of inorganic cations and ammonium in municipal wastewater.
Figure 3 – Determination of trace ammonium in the presence of high sodium (1:10,000).Conclusion
Compact, high-pressure ion chromatography systems equipped with high-capacity 4-μm particle cation-exchange columns are highly efficient for the analysis of inorganic cations and ammonium in environmental waters, facilitating adherence to current U.S., EU and Japanese waterquality recommendations and regulations. The high pressure capability allowed use of a flow rate of 0.9 mL/min, reducing run time considerably and increasing sample throughput, while providing precise, reliable and reproducible results. A wide range of environmental waters can be monitored using these columns, while resolving trace ammonium in the presence of high sodium concentrations.
References
- World Health Organization. pH in Drinking Water. Background Document for Development of WHO Guidelines for Drinking-Water Quality; Geneva, Switzerland, 2003.
- World Health Organization. Hardness in Drinking Water. Background Document for Development of WHO Guidelines for Drinking-Water Quality; Geneva, Switzerland, 2011.
- Water Research Center. Ammonia in Groundwater, Runoff, and Streams; http://www.water-research.net/index.php/ammonia-ingroundwater-runoff-and-streams
- U.S. Environmental Protection Agency. Aquatic Life Criteria—Ammonia https://www.epa.gov/wqc/aquatic-life-criteria-ammonia
- Council Directive 98/83/EC of 3 November 1998 on the quality of water intended for human consumption.
- Ministry of the Environment, Government of Japan. National Effluent Standards; https://www.env.go.jp/en/water/wq/nes.html
- Michalski, R. Applications of ion chromatography for the determination of inorganic cations. Crit. Rev. Anal. Chem. 2009, 39(4), 230–50.
- American Public Health Association. Standard Methods for the Analysis of Water and Wastewater, 18th edition, pp 4-75 to 4-85; Washington, D.C., 1992.
- Jackson, P.E. Ion Chromatography in Environmental Analysis, Encyclopedia of Analytical Chemistry; Meyers, R.A., Ed.; John Wiley & Sons; Chichester, U.K., 2000, pp 2779–2801.
- U.S. EPA Method 300.7. Dissolved Sodium, Ammonium, Potassium, Magnesium, and Calcium in Wet Deposition by Chemically Suppressed Ion Chromatography; U.S. EPA, Cincinnati, Ohio, 1986.
- ISO 14911. 1998 Water Quality—Determination of Dissolved Li+, Na+, NH4+, K+, Mn2+, Ca2+, Mg2+, Sr2+ and Ba2+ Using Ion Chromatography; Method for Water and Wastewater.
- ASTM D6919–09. Standard Test Method for Determination of Dissolved Alkali and Alkaline Earth Cations and Ammonium in Water and Wastewater by Ion Chromatography; ASTM International, West Conshohocken, Penn., 2009.
Carl Fisher is application scientist and senior marketing specialist, Thermo Fisher Scientific, 490 Lakeside Dr., Sunnyvale, Calif. 94085, U.S.A.; tel.: 408-737- 0700; e-mail: [email protected]; www.thermofisher.com