Gas chromatography/mass spectrometry has made it possible to run large numbers of components in a single sample injection. The GC/MS methods introduced in the late 1960s and early 1970s for environmental compliance and monitoring were superior to previous GC detection methods because the mass selective detector enabled both quantitation and confirmation without second column confirmation. However, even with capillary columns, these methods still suffered from coelution, limiting the number of components or requiring long analysis times. Fortunately, advances in GC/MS technology have helped to overcome these limitations, enabling the analysis of hundreds of compounds in a fraction of the time.
Gas chromatography separates components in a mixture
GC is used to separate mixtures of compounds by partitioning between two phases. One phase is stationary, with a large surface area, and the other is a gas that travels through or across the stationary phase. Sample components in the mixture are vaporized and carried by the gas phase through a column containing the stationary phase. As the components contact the stationary phase, they are adsorbed onto its surface as a function of solubility and temperature. The components separate from one another during the process.
A gas chromatograph consists of an injector to vaporize the sample, a column that contains the stationary phase and a detector that responds to some physical property of the sample components to produce a signal. The result is a chromatogram that plots the elution of the individual components with time versus intensity. The time the component elutes—called the retention time—identifies the compound, and the intensity is proportional to the concentration. While the detectors used may correspond to specific characteristics of the sample (for example, the sample contains halogens), a GC detector does not provide confirmation that the compound eluting at that particular retention time is the compound one thinks it is, nor can it guarantee that the peak one sees is not a combination of several compounds eluting together; GC methods do not provide qualitative identification.
GC/MS measures and identifies the components in a mixture
A gas chromatograph coupled with a mass spectrometer replaces the nonselective detectors of traditional gas chromatography with a mass selective detector. Mass spectrometry detection provides GC analysis with a means to obtain qualitative and quantitative information. The eluting compounds are ionized and fragmented. The positively charged fragments are propelled into a mass detector that separates them by mass into a distinctive and highly reproducible fragmentation pattern (see Figure 1 for an example of GC/MS spectra). Benchtop GC/MS typically uses a single quadrupole, ion trap or time-of-flight mass spectrometer. Most GC/MS systems in use today are quadrupoles because they are smaller, less costly and capable of scanning across the entire mass range at thousands of scans per second.
Figure 1 – Example of the mass spectra of caffeine.Operation of the single quadrupole mass spectrometer
A single quadrupole GC/MS can be operated in two modes—either as a total ion current (TIC) or as a small number of individual ions at fixed retention time windows (selected ion monitoring, SIM). TIC mode, also called full scan mode, is necessary for the qualitative identification of unknowns and confirmation of targeted compounds. In full scan mode, a specific range (e.g., 50–550 amu) is scanned at a rate fast enough to collect 10–20 scans per peak. Mass spectra data are collected for all peaks and to determine the baseline between the peaks. Peak spectra are compared to previously injected standards or to a spectral library, such as an NIST database.
In SIM mode, only a few ions are monitored at the target ion retention window. For example, suppose a peak is 6 seconds wide. A GC/MS full scan method may be set to scan a mass range of 50–550 amu at 3 scans per second. This will yield 18 scans across the peak, enabling good full scan spectra and quantitation. To do this, the MS is set to scan at 0.3 scans per second. In SIM mode, however, only 1 amu will be scanned as opposed to a full range. Therefore, the entire 0.3 seconds will be spent measuring that one mass. Because only one mass is monitored for a longer period of time, the sensitivity increases, lowering the detection limit. But, since only one mass is monitored, SIM data cannot be used for qualitative confirmation.
Even though SIM is highly selective, it is still possible for ions of the same mass to coelute, particularly in highly complex matrices. Colliding the SIM ion into an inert gas, such as argon, fragments the SIM into product ions that can be separated in yet another mass selective detector and effectively eliminate all matrix noise and coeluting interference. The combination of SIM→ collision→ SIM is termed multiple reaction monitoring (MRM) and is the technique applied on triple quadrupole (or tandem) GC/MS analyzers.
Tandem GC/MS removes interferences and separates peaks by mass
Tandem mass spectrometry is the linking of two quadrupoles. Ions are separated in the first quadrupole and fragmented further in a collision cell, and the product ions are separated and analyzed by a second quadrupole. (Although these analyzers are called triple quadrupole analyzers, there are technically only two quadrupoles with a collision cell between them.) This technique eliminates matrix interferences, making it highly selective and extremely sensitive. Data can be collected in at least four modes, as shown in Table 1. The faster scanning analyzers are also capable of scan/MRM and scan/SIM all in a single method.
Table 1 – Analysis modes for tandem GC/MS analyzers
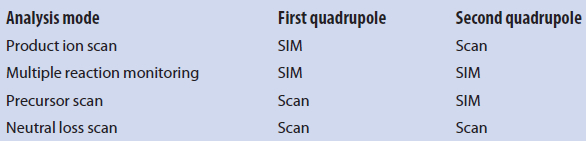
MRM is used for maximum selectivity and the lowest detection limits. The technique eliminates matrix noise and allows only the selected product ion through the second quadrupole.
Although the sensitivity is actually less, the noise is reduced significantly compared to SIM increasing the signal-to-noise ratio. Figure 2 is a schematic of tandem quadrupoles and how noise is eliminated. In Figure 3, SIM and MRM chromatograms show the decrease in noise and signal and the corresponding increase in the MRM signal-to-noise ratio. MRM detection limits are typically 5–10 times lower than SIM detection limits.
Figure 2 – Schematic of a triple quadrupole analyzer and how noise is eliminated.
Figure 3 – Example chromatogram showing increased MRM signal-to-noise.Tandem GC/MS shortens analysis times, increasing laboratory productivity
The important and often overlooked aspect of tandem GC/MS is the ability to separate compounds by mass. Unlike the gas chromatography method with nonselective detectors mentioned above, triple quadrupole GC/MS allows reliable detection of coeluting compounds. Figure 4 is an example of a chromatogram of over 400 target compounds, many of which are coeluting. The MRM technique is so selective that it separates these coeluting compounds by mass within the detector.
Figure 4 – Chromatogram of over 400 pesticides.Chromatography no longer needs to separate all compounds on the column. With new tandem GC/MS instruments, it is now possible to selectively analyze hundreds of compounds with very low detection limits in a fraction of the time possible by GC or single quadrupole GC/MS.
Conclusion
Gas chromatography is a technique used to separate and quantitate components in a mixture. Coupled with mass spectrometry, it is a powerful technique that provides both qualitative verification of the identity of a chromatographic peak and quantitation. Selected ion monitoring increases the sensitivity of GC/MS methods but loses the qualitative functionality. New GC/MS analyzers link two quadrupole mass selective detectors together. A collision cell between the two quadrupoles fragments ions emerging from the first quadrupole into product ions, eliminating noise and enabling extremely sensitive and highly specific target compound analysis. These new GC/MS analyzers are so specific that chromatography methods no longer need to separate all compounds, allowing the accurate quantitation of hundreds of peaks within minutes.
William Lipps is environmental/chemical business unit manager, Shimadzu Scientific Instruments, Inc., 7102 Riverwood Dr., Columbia, Md. 21046, U.S.A.; tel.: 410- 381-1227; e-mail: [email protected]; www.shimadzu.com