Ultrahigh-speed liquid chromatography-mass spectroscopy instruments can accelerate analysis times by at least tenfold compared to conventional LC-MS-MS systems. Recently, there has been a question in the research community as to whether conventional LC-MS-MS systems can attain ultrahigh-speed analysis by using a sub-2-μm column.
To answer this question, researchers must address the challenges associated with conventional LC-MS-MS systems when attempting ultrahigh-speed analysis. These issues include separation deterioration, a reduced number of acquired points, and increased cross-talk. This article offers methods to resolve these problems and to help achieve ultrahigh-speed analysis.
Separation deterioration
When attempting ultrahigh-speed analysis, first consider the LC instrument itself. Shortened analysis times can be achieved by reducing the column volume (in other words, shortening the length) or by increasing the mobile phase flow rate. However, separation will deteriorate with a typical column.
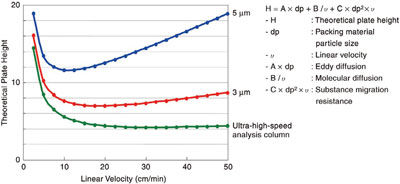
Figure 1 - van Deemter theory.
A better approach is to reduce the column particle size and increase the number of theoretical plates. Sub-2-μm particle sizes are now used worldwide. Because there is no change in theoretical plate height when the flow rate is increased, separation is maintained (Figure 1). However, if a sub-2-μm column is installed in an LC instrument that was not initially designed for ultrahigh-speed analysis, instead of behaving according to theory, the separation lacks optimization notwithstanding the shortened analysis time. This results from the relative increase in diffusion within the LC instrument flow line.
In some cases, maximized LC separation is not necessary because MS-MS selectivity is high. However, separation from impurities is frequently required in order to ensure data reliability. It is therefore preferable to maximize the resolution of any LC separation.
Most importantly, a significant increase in pressure is associated with sub-2-μm separations. Because the pressure tolerance will be exceeded in LC instruments not designed for ultrahigh speeds, sub-2-μm columns cannot be used.
Reduced number of acquired points
When ultrahigh-speed analysis is conducted using ultrahigh-performance liquid chromatography (UHPLC), peaks entering the MS are extremely narrow. However, with conventional MS-MS instruments that cannot handle ultrahigh speeds, duty cycles are long and acquisition rates are slow. This results in a reduced number of points across each peak.
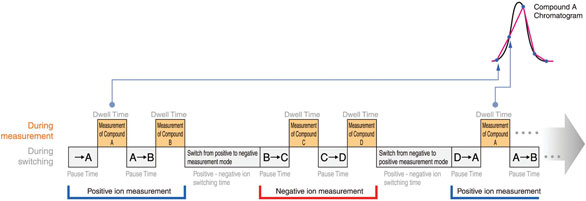
Figure 2 - Example of MRM measurement while positive-negative ion switching between four compounds A–D.
These MS-MS instruments squander time while changing analysis conditions such as positive-negative ion switching or multiple reaction monitoring (MRM) transitions, reducing the time available for the real work of acquiring data (Figure 2). For instance, when using a remote control to switch from one channel to another on television, program viewing is momentarily unavailable. Similarly, no ionization is conducted during positive-negative ion switching time in an MS-MS. Also, when compound B is to be measured following compound A, measurement does not take place during the MRM switching operation (pause time). Therefore, the longer the time required for switching, the fewer the number of points acquired.
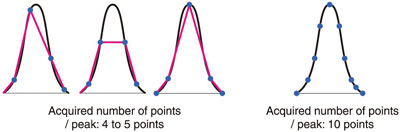
Figure 3 - Relationship between acquired point number and chromatogram peak shape.
The number of data points acquired has significant bearing on the accuracy of the peak shapes generated. A smaller number of points results in non-Gaussian, nonrepeatable peak shapes. Figure 3 shows the relationship between the number of acquired points and chromatogram shape. It is clear that at least 10 data points per peak are required to obtain reproducible, smooth peak shapes.
When the number of acquired points per peak is only four or five, there is a large divergence from a true peak shape. Not only does peak-shape distortion occur, but the repeatability of the chromatogram itself is diminished. This adversely affects the quantitation results and sensitivity.
Increased cross-talk
In conventional MS-MS instruments that cannot support ultrahigh speed, the compound ions stall and lose speed in the collision cell, which increases cross-talk. The product ions generated through collision with the inert gas in the collision cell travel toward the exit of the collision cell, but because ions slow down during this process, part of this migration toward the exit is still ongoing even after the next dwell time (measurement) starts.
When these residual product ions pass Q3 and reach the detector, they are erroneously detected as product ions of the ongoing measurement. This phenomenon is referred to as cross-talk, and it adversely affects the qualitative and quantitative results as well as sensitivity. In instruments that are not designed for high-speed analysis, cross-talk is amplified as the duty cycle decreases.
Resolving the problems
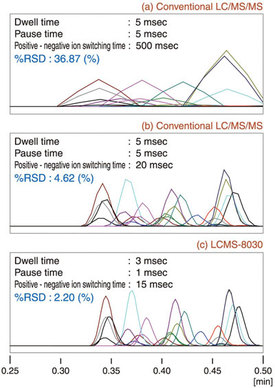
Figure 4 - Positive-negative ion switching and MRM measurement ultrahigh-speed test.
In response to the widespread use of UHPLC together with the demand for ultrahigh-speed LC-MS-MS, an MS-MS instrument (LCMS- 8030, Shimadzu Corp., Kyoto, Japan) has been developed that demonstrates ultrahigh-speed performance in combination with UHPLC. As shown in Figure 4, the instrument achieves ultrahigh-speed analysis that has not been possible with previous LC-MS-MS instruments.
Below are seven requirements for an ultrahigh-speed LC-MS-MS. These have now been addressed.
Ultrahigh-speed gradient
Ultrahigh-speed, high-accuracy gradient delivery is achieved with a solvent delivery pump that is able to deliver up to 3 mL/min at its maximum pressure rating (130 MPa: 0.0001–3 mL/ min, 80 MPa: 3.0001–5 mL/min) together with a 20-μL ultrahigh-efficiency mixer.
Ultrahigh-speed injection
A system that can merely complete a single analysis in a short time cannot be said to support true ultrahigh-speed analysis. The total cycle time must be considered. A UHPLC equipped with an autosampler that has a newly improved injection unit can achieve low carryover even without time-consuming needle rinsing. With an injection taking barely 10 sec, ultrahigh-speed analysis is possible because long autosampler cycle times are eliminated.