In all too many applications of gas chromatography (GC), there is a need for supporting confirmation of a result. Retention time alone is simply not definitive enough. There are many options, including using a secondary confirmatory GC column, which may require running a sample again. While this may be practical, it is time consuming. Often one must change the column or use a separate, dedicated instrument. If the need is infrequent, then starting up the confirmatory gas chromatograph will be time consuming. The startup time often extends the analysis time to the next shift.
For these reasons, many laboratories in the developed world have opted to use a mass spectrometer (MS) as the secondary or confirmatory detector, especially in GC. Indeed, when MS is suitable, it is a fine detection technology. However, the acquisition and running cost are high. Plus, the analyst needs to be proficient in interpreting the idiosyncrasies of the ion sources and the impact on GC-MS spectra. This often means that the lab requires a GC-MS specialist, which also has an associated higher cost. MS instruments do benefit from experienced operators and service staff. For this reason, many vendors recommend a service interval as short as two months.
Table 1 – Selective detectors for gas chromatography (excluding MS)* (source: vendor interviews and literature)
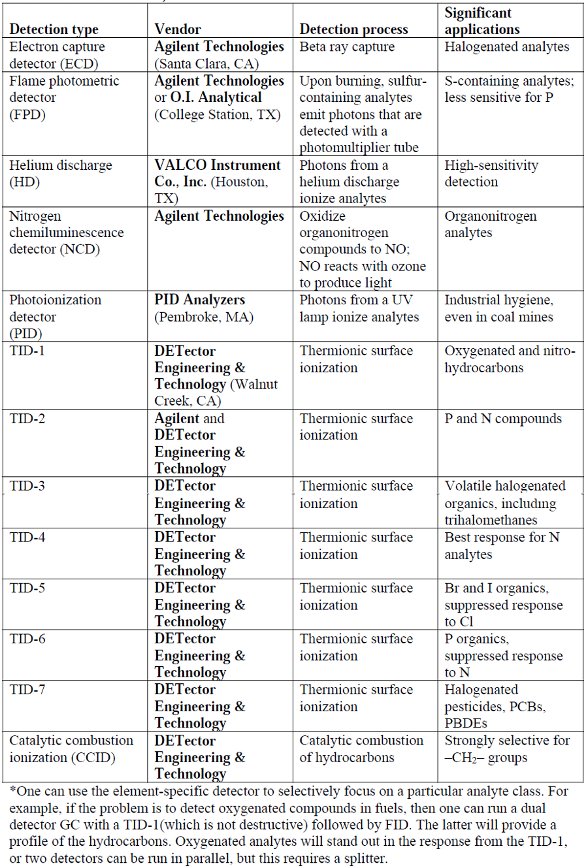
Rather than focusing on MS detection, experienced gas chromatographers can select from a list of highly discriminating detectors for GC to supplement and thus confirm results from the flame ionization detector (FID) and thermal conductivity detector (TCD). Some detector options are presented in Table 1. In many cases, the element-specific detector might be sufficient, since the analytes of interest may all have an element in common, other than carbon and hydrogen, of course. For example, if the analytes of concern are all halocarbons such as polychlorinated biphenyls (PCBs), then one could select a detector such as the TID-7 from DETector Engineering & Technology and really simplify the chromatography.
How GC detectors operate
Helium ionization–Pulsed discharge detector
The pulsed discharge detector operates in two modes, both of which provide opportunities for selective detector tuning. In the electron capture mode, the compounds with a high electron affinity such as halocarbons entering the detection cell decrease the standing current. Typical detection limits fall in the range of 10–15 to 10–12 g. In pulsed discharge mode, high-energy photons from a helium discharge ionize the analytes. The liberated electrons cause changes in the standing current, which are amplified for the detector signal. Detection sensitivity is superior to conventional sources such as 63Ni.
Electron capture detector
Electron capture detectors qualify as the classic selective detector, having been invented in the late 1950s. A radioisotope such as 3H or 63Ni is placed in a cell with a collection anode. In the absence of analyte, a small, finite “standing” current flows from the beta ray source to the collection anode. However, when analytes with high electroaffinity are present, the electrons are captured, which reduces the standing current. The loss of current is amplified and displayed as the response.
Photoionization detector
Photoionization detectors are conceptually similar to the pulsed discharge detector, except that the UV light directly illuminates the content of the detector cell. The light ionizes all molecules that have an ionization energy equal to or less than the energy of the light emitted from the lamp. The electrons are attracted to the anode and the resulting current is amplified for the detector output.
Flame photometric detector
Flame photometric detectors burn the column effluent in a flame. Photons from the flame are converted to an electric current with a photomultiplier tube. The detection limit for sulfur is about 4 pg/sec. Detection of phosphorus is more than 10 times worse. Nitrogen chemiluminesnce detection relies on the reaction of NO with ozone to produce a photon that is measured with a photomultiplier. These detectors are quite popular since they are so specific to the elements. They are moderately expensive, however.
Thermionic surface ionization
Thermionic surface ionization describes a class of chromatographic transducers that utilize hot ceramic surfaces where small differences in work function produce element-specific ionization.1 These detectors (TID-1 through TID-7 [Table 1]) differ by the composition of the active surface. Small ceramic beads are heated in a detector that resembles a cell for a flame ionization detector, but without the flame. The column effluent passes over the hot surface. Analytes that possess the right functional group for the bead, contact the surface, and are ionized.2 Ionization changes the standing current passing through the cell, which is amplified as with an FID. Typically, the lifetime of the ceramic bead is a few years. Beads are easily replaced and recycled. The chromatographer can identify peaks based on the retention time and relative response (response ratio) of the peaks detected by a universal detector such as an FID and an element-specific detector. The price of a typical thermionic detector is less than 5% of the cost of an MS detector. Plus, maintenance is easier and less demanding.
Group-specific detector
Catalytic combustion ionization detection (CCID) is the newest entry in the table. This is a novel detector that responds preferentially to –CH2– groups. Column effluent containing combustible analytes enters an oxygen-rich detector similar to an FID. The detector cell also contains a hot catalytic surface. The mixture ignites and produces a thermionic emission that is detected in a manner similar to the TID series of detectors from DETector Engineering & Technology. This detector responds very strongly to methylene groups compared to other hydrocarbon structures. Detection sensitivity for –C=C– is low, and –C≡C– is even weaker. Thus, the CCID can be used to discriminate between isomers such as alkylbenzenes.
Conclusion
If element-specific detectors are so good, why is GC-MS so popular? MS does provide more information, especially if fragmentation patterns are considered. But this information is seldom used in practice, since the analytes are usually well known and can be identified by comparing the retention time with standards. MS achieved its popularity 20 years before the thermionic detectors became easily available. Today’s preference for MS would probably be quite different if the thermionic detection preceded the introduction of GC-MS. Element- and group-specific detectors save money, are easy to use, and allow people with less training to make good decisions. This is what separation science is all about.
References
- Vijaya Saradhi, U.; Suryanarayana, M. et al. J. Chromatogr. A 2001, 911(1): 63–73.
- Patterson, P. Nitrogen Phosphorous Detector. In: Detectors for Capillary Chromatography; Hill, H.; McMinn, D., Eds.; John Wiley & Sons: New York, NY, 1992; pp 139–66.
Robert L. Stevenson, Ph.D., is a Consultant and Editor of Separation Science for American Laboratory/Labcompare; e-mail: [email protected].